In the fascinating world of biology, transcription is a crucial process that bridges the gap between DNA and RNA, serving as an essential step in the central dogma of molecular biology. Understanding where transcription takes place is key to grasping the intricate processes that sustain life at a cellular level. This article delves deeply into the cellular environment of transcription, exploring its location, mechanics, and significance in both prokaryotic and eukaryotic organisms. Across the vast diversity of life, transcription occurs in specific cellular compartments, each tailored to facilitate this complex process. By comprehending the nuances of where transcription takes place, we gain insights into cellular function and regulation, which have profound implications for fields such as genetics, medicine, and biotechnology.
In this article, we will explore various aspects of transcription, including its definition and significance, the intricate machinery involved, and the precise locations within the cell where it takes place. We will also examine the differences and similarities in transcription between prokaryotic and eukaryotic cells, shedding light on the evolutionary adaptations that have shaped these processes. By the end of this comprehensive guide, you will have a clear understanding of where transcription occurs and why it is fundamental to all living organisms.
The exploration of transcription is not just a journey into the microscopic world of cells, but also a journey into the very essence of life itself. As we navigate through the various sections of this article, we will uncover the remarkable complexity and elegance of transcription, revealing how this process underpins the genetic blueprint that defines every living organism. Whether you are a student, educator, or simply a curious reader, this guide aims to provide a thorough and engaging exploration of where transcription takes place and its critical role in the continuity of life.
Table of Contents
- Definition and Significance of Transcription
- Cellular Machinery Involved in Transcription
- Where Does Transcription Take Place in Prokaryotes
- Where Does Transcription Take Place in Eukaryotes
- Role of Nucleus in Eukaryotic Transcription
- Cytoplasmic Transcription
- Mitochondrial Transcription
- Chloroplast Transcription in Plants
- Comparison Between Prokaryotic and Eukaryotic Transcription
- Regulation of Transcription
- Transcription Factors and Their Role
- Impact of Transcription Errors
- Transcription in Disease and Medicine
- Biotechnological Applications of Transcription
- Future Directions in Transcription Research
- Frequently Asked Questions
- Conclusion
Definition and Significance of Transcription
Transcription is the process by which genetic information encoded in DNA is transcribed into messenger RNA (mRNA) molecules. This process is pivotal in the central dogma of molecular biology, which describes the flow of genetic information within a biological system. It serves as the first step in the expression of genes, ultimately leading to the synthesis of proteins that perform various functions necessary for the survival and maintenance of living organisms.
The significance of transcription lies in its ability to accurately copy genetic information, ensuring that the correct proteins are produced at the right time and in the right amounts. This precise regulation is essential for cellular function and adaptation to changing environments. Any errors in transcription can lead to significant consequences, including genetic diseases and developmental abnormalities.
Moreover, transcription is a highly regulated process that allows cells to respond dynamically to external signals and internal cues. This adaptability is crucial for processes such as cell differentiation, growth, and immune response. Understanding transcription and its regulation provides valuable insights into the mechanisms that underpin cellular life and the basis of many diseases.
Cellular Machinery Involved in Transcription
The transcription process involves a complex assembly of proteins and enzymes, collectively referred to as the transcription machinery. Central to this process is the enzyme RNA polymerase, which catalyzes the synthesis of RNA by reading the DNA template. In prokaryotic cells, a single type of RNA polymerase is responsible for transcribing all types of RNA, whereas eukaryotic cells have three distinct RNA polymerases, each dedicated to transcribing different classes of RNA.
In addition to RNA polymerase, various transcription factors play a crucial role in initiating and regulating transcription. These factors bind to specific DNA sequences near gene promoters, facilitating the recruitment of RNA polymerase and the formation of the transcription initiation complex. Other proteins, such as co-activators and co-repressors, modulate the activity of transcription factors, fine-tuning the transcriptional response.
The transcription machinery also includes several key components involved in RNA processing and modification. These include capping enzymes, spliceosomes, and polyadenylation factors, which ensure that the transcribed RNA is properly processed and matured before being exported from the nucleus for translation.
Where Does Transcription Take Place in Prokaryotes
In prokaryotic organisms, such as bacteria, transcription occurs in the cytoplasm. Unlike eukaryotic cells, prokaryotes lack a defined nucleus, allowing transcription and translation to occur simultaneously. This spatial and temporal coupling of transcription and translation is a distinctive feature of prokaryotic gene expression, enabling rapid responses to environmental changes.
The transcription process in prokaryotes begins when RNA polymerase binds to the promoter region of a gene. The promoter is a specific DNA sequence that signals the start of transcription. Once bound, RNA polymerase unwinds the DNA and begins synthesizing RNA by adding ribonucleotides complementary to the DNA template strand.
Prokaryotic transcription is characterized by the simplicity and efficiency of its regulatory mechanisms. Operons, which are clusters of genes transcribed together as a single mRNA molecule, provide a streamlined means of coordinating gene expression. Regulatory proteins, such as repressors and activators, modulate the activity of operons by binding to operator sequences, influencing the binding of RNA polymerase to the promoter.
Where Does Transcription Take Place in Eukaryotes
In eukaryotic cells, transcription takes place primarily in the cell nucleus. The compartmentalization of transcription within the nucleus allows for greater regulation and complexity in gene expression compared to prokaryotes. Eukaryotic transcription involves multiple steps and a highly coordinated interaction between various molecular components.
The process begins with the binding of transcription factors to specific promoter sequences upstream of a gene. These factors recruit RNA polymerase II, the enzyme responsible for transcribing mRNA, to form the transcription initiation complex. Once assembled, RNA polymerase II proceeds to unwind the DNA and synthesize RNA by elongating the RNA strand in the 5' to 3' direction.
In addition to the nucleus, eukaryotic cells also contain other organelles, such as mitochondria and chloroplasts, which have their own transcription machinery. Mitochondrial and chloroplast transcription are distinct processes that occur within these organelles, reflecting their evolutionary origins as symbiotic bacteria.
Role of Nucleus in Eukaryotic Transcription
The nucleus plays a central role in eukaryotic transcription by providing a specialized environment for the transcription and processing of RNA. As the site of chromatin organization, the nucleus contains the genetic material in the form of chromatin, which is a complex of DNA and histone proteins. The dynamic organization of chromatin influences the accessibility of genes to the transcription machinery.
The nuclear membrane serves as a barrier, separating transcription from translation, which occurs in the cytoplasm. This separation allows for extensive RNA processing, including capping, splicing, and polyadenylation, to occur before the mature mRNA is exported to the cytoplasm for translation. The nuclear envelope also contains nuclear pores, which regulate the transport of RNA and proteins between the nucleus and cytoplasm.
Moreover, the nucleus is home to various nuclear bodies, such as nucleoli and speckles, which are involved in specific aspects of RNA processing and ribosome biogenesis. These subnuclear structures contribute to the organization and efficiency of transcriptional and post-transcriptional processes.
Cytoplasmic Transcription
While the majority of transcription in eukaryotic cells occurs in the nucleus, certain types of transcription can also take place in the cytoplasm. This includes the transcription of mitochondrial and chloroplast genes, which are transcribed within the organelles themselves. These organelles contain their own DNA and transcription machinery, reflecting their evolutionary history as independent prokaryotic organisms.
Mitochondrial transcription involves a single RNA polymerase that synthesizes RNA from the mitochondrial DNA. This process is essential for the production of mitochondrial proteins required for oxidative phosphorylation and energy production. Similarly, chloroplast transcription is responsible for the synthesis of RNA from chloroplast DNA, which is crucial for photosynthesis and other plastid functions.
The cytoplasmic transcription of organellar genes highlights the complexity and diversity of transcriptional processes in eukaryotic cells. It underscores the importance of organelle-specific transcription in maintaining cellular and metabolic functions.
Mitochondrial Transcription
Mitochondrial transcription is a specialized process that occurs within the mitochondria, an organelle known as the powerhouse of the cell. Mitochondria possess their own circular DNA, distinct from the nuclear DNA, and are equipped with the machinery necessary for transcribing this genetic material. The process is orchestrated by a single mitochondrial RNA polymerase, along with other essential transcription factors and proteins.
The transcription of mitochondrial genes is crucial for the production of proteins involved in the electron transport chain, a series of complexes that generate ATP, the cell's primary energy currency. Mitochondrial transcription also produces transfer RNAs (tRNAs) and ribosomal RNAs (rRNAs), which are integral to mitochondrial protein synthesis.
Regulation of mitochondrial transcription is tightly controlled and responsive to the energy demands of the cell. Factors such as mitochondrial transcription factor A (TFAM) play a pivotal role in regulating the transcription of mitochondrial DNA by binding to promoters and initiating transcription. The coordination between mitochondrial and nuclear transcription is essential for maintaining cellular energy balance and function.
Chloroplast Transcription in Plants
Chloroplast transcription is a vital process occurring within chloroplasts, the organelles responsible for photosynthesis in plant cells. Like mitochondria, chloroplasts have their own circular DNA and transcription machinery, reflecting their origin as endosymbiotic cyanobacteria. Chloroplast transcription is essential for the synthesis of proteins required for photosynthesis and other chloroplast functions.
The transcription machinery in chloroplasts includes a unique RNA polymerase, along with a variety of transcription factors and regulatory proteins. Chloroplast transcription is regulated by light, developmental cues, and environmental factors, ensuring that chloroplast genes are expressed in response to the needs of the plant.
Chloroplast transcription contributes to the biogenesis and maintenance of the photosynthetic apparatus, enabling plants to capture light energy and convert it into chemical energy. The coordination of chloroplast and nuclear transcription is crucial for the proper assembly and function of the photosynthetic machinery.
Comparison Between Prokaryotic and Eukaryotic Transcription
While the fundamental principles of transcription are conserved across prokaryotic and eukaryotic organisms, there are notable differences in the mechanisms and regulation of this process. These differences reflect the distinct cellular architecture and complexity of prokaryotic and eukaryotic cells.
In prokaryotes, transcription occurs in the cytoplasm, allowing for the simultaneous transcription and translation of genes. This coupling enables rapid responses to changing environmental conditions, a key feature of prokaryotic gene expression. Prokaryotic transcription is relatively simple, with a single RNA polymerase responsible for transcribing all classes of RNA.
In contrast, eukaryotic transcription takes place primarily in the nucleus, with transcription and translation separated temporally and spatially. Eukaryotic cells possess multiple RNA polymerases, each dedicated to transcribing specific types of RNA. The complexity of eukaryotic transcription is further increased by the presence of introns in many genes, requiring extensive RNA processing and splicing.
Eukaryotic transcription is also subject to a higher degree of regulation, involving a multitude of transcription factors, co-activators, and chromatin modifications. This regulation allows for precise control of gene expression in response to developmental signals and environmental stimuli.
Regulation of Transcription
The regulation of transcription is a sophisticated process that ensures genes are expressed at the right time, in the right cell type, and in the appropriate amounts. This regulation is achieved through a combination of genetic, epigenetic, and environmental factors that influence the activity of transcription machinery.
One of the primary means of transcriptional regulation is through the binding of transcription factors to specific DNA sequences near the promoter region of a gene. These factors can either activate or repress transcription by facilitating or inhibiting the recruitment of RNA polymerase. The activity of transcription factors is modulated by various signals, such as hormones, growth factors, and stress, which allow cells to adapt to changing conditions.
Epigenetic modifications, such as DNA methylation and histone acetylation, also play a crucial role in regulating transcription. These modifications alter the accessibility of chromatin to the transcription machinery, influencing the expression of genes without changing the underlying DNA sequence. Epigenetic regulation is essential for processes such as development, differentiation, and response to environmental cues.
Transcription Factors and Their Role
Transcription factors are proteins that play a pivotal role in regulating gene expression by binding to specific DNA sequences near promoters and enhancers. They act as molecular switches, turning genes on or off in response to various signals and stimuli. Transcription factors can function as activators, enhancing gene expression, or as repressors, inhibiting gene expression.
The activity of transcription factors is regulated by several mechanisms, including post-translational modifications, interactions with co-factors, and changes in subcellular localization. These regulatory mechanisms allow transcription factors to respond dynamically to cellular and environmental signals, ensuring precise control of gene expression.
Transcription factors are involved in a wide range of biological processes, including development, cell differentiation, immune response, and metabolism. Their dysregulation can lead to various diseases, including cancer, autoimmune disorders, and developmental abnormalities. Understanding the function and regulation of transcription factors is crucial for developing therapeutic strategies targeting transcriptional dysregulation.
Impact of Transcription Errors
Transcription errors, although relatively rare, can have significant consequences for cellular function and organismal health. Errors in transcription can result in the production of faulty or aberrant RNA molecules, leading to the synthesis of dysfunctional proteins. These errors can arise from various sources, including mutations, environmental stress, and defects in the transcription machinery.
The impact of transcription errors depends on the nature and location of the error. Errors in critical regions of a gene can disrupt protein function, leading to diseases such as cancer, neurodegenerative disorders, and metabolic syndromes. In some cases, transcription errors can trigger cellular stress responses, such as the unfolded protein response, which aims to alleviate the effects of faulty protein production.
Cells have evolved mechanisms to minimize and correct transcription errors, including proofreading by RNA polymerase and quality control pathways that degrade aberrant RNA. Understanding the causes and consequences of transcription errors is important for elucidating the molecular basis of diseases and developing strategies to mitigate their effects.
Transcription in Disease and Medicine
Transcription plays a critical role in the development and progression of various diseases, making it a key focus of medical research and therapeutic interventions. Dysregulation of transcription can lead to the aberrant expression of genes, contributing to conditions such as cancer, cardiovascular diseases, and neurodegenerative disorders.
Cancer is a prime example of a disease associated with transcriptional dysregulation. Mutations in transcription factors or co-factors can lead to uncontrolled cell proliferation, evasion of apoptosis, and metastasis. Targeting transcriptional pathways has become a promising strategy in cancer therapy, with drugs designed to inhibit or modulate the activity of specific transcription factors.
In addition to cancer, transcriptional dysregulation is implicated in various other diseases, including autoimmune disorders, metabolic syndromes, and genetic diseases. Understanding the transcriptional changes associated with these conditions provides valuable insights into their pathogenesis and potential therapeutic targets.
Advancements in transcription-related research have led to the development of novel therapeutic approaches, such as gene therapy, RNA-based therapies, and small molecule inhibitors. These approaches aim to restore normal transcriptional regulation and ameliorate disease symptoms.
Biotechnological Applications of Transcription
The understanding and manipulation of transcription have numerous applications in biotechnology, with implications for research, medicine, and industry. One of the most significant applications is the use of transcription-based technologies in genetic engineering and synthetic biology.
Transcriptional regulation is harnessed in the development of gene expression systems for the production of recombinant proteins, vaccines, and therapeutic agents. By controlling the transcription of specific genes, researchers can optimize protein production for various applications, including pharmaceuticals, biofuels, and industrial enzymes.
In synthetic biology, transcriptional circuits are engineered to create novel biological systems with desired functions. These circuits can be used to develop biosensors, bioreactors, and other biotechnological tools with applications in environmental monitoring, agriculture, and healthcare.
Moreover, transcriptional profiling and RNA sequencing are powerful techniques used to study gene expression patterns and identify biomarkers for disease diagnosis and prognosis. These technologies have revolutionized our understanding of transcriptional regulation and its role in health and disease.
Future Directions in Transcription Research
Transcription research is a rapidly evolving field, with ongoing advancements in technology and methodology driving new discoveries. Future directions in transcription research are likely to focus on several key areas, including the elucidation of complex regulatory networks, the development of novel therapeutic approaches, and the application of transcriptional insights to biotechnology.
One promising area of research is the study of non-coding RNAs and their role in regulating transcription and gene expression. Non-coding RNAs, such as microRNAs and long non-coding RNAs, have emerged as important regulators of transcriptional processes, with implications for development and disease.
Another area of interest is the integration of transcriptional data with other omics data, such as genomics, proteomics, and metabolomics, to gain a comprehensive understanding of cellular function and regulation. This systems biology approach has the potential to uncover novel regulatory mechanisms and identify new therapeutic targets.
Advancements in gene editing technologies, such as CRISPR-Cas9, offer exciting opportunities for manipulating transcriptional regulation in a precise and targeted manner. These technologies hold promise for developing new treatments for genetic diseases and for engineering cells with enhanced capabilities.
Frequently Asked Questions
1. What is transcription, and why is it important?
Transcription is the process of copying genetic information from DNA into RNA. It is essential for gene expression, allowing cells to produce proteins necessary for their function and survival.
2. Where does transcription take place in prokaryotic cells?
In prokaryotic cells, transcription occurs in the cytoplasm, where it is coupled with translation for efficient gene expression.
3. How does transcription differ between prokaryotic and eukaryotic cells?
Transcription in prokaryotic cells occurs in the cytoplasm, with a single RNA polymerase transcribing all RNA types. In eukaryotic cells, transcription occurs in the nucleus, involves multiple RNA polymerases, and includes extensive RNA processing.
4. What role do transcription factors play in gene expression?
Transcription factors regulate gene expression by binding to specific DNA sequences, modulating the recruitment of RNA polymerase, and responding to cellular and environmental signals.
5. How do transcription errors affect cellular function?
Transcription errors can lead to the production of faulty proteins, potentially causing diseases such as cancer and metabolic disorders. Cells have mechanisms to minimize and correct these errors.
6. What are some biotechnological applications of transcription?
Transcription-based technologies are used in genetic engineering, synthetic biology, and biotechnology for the production of recombinant proteins, development of biosensors, and transcriptional profiling for disease diagnosis.
Conclusion
Transcription is a fundamental biological process that plays a critical role in the expression of genetic information and the regulation of cellular functions. Understanding where transcription takes place provides valuable insights into the complexity and diversity of life at a cellular level. From the cytoplasm of prokaryotic cells to the nucleus and organelles of eukaryotic cells, transcription occurs in specific cellular compartments tailored to facilitate this intricate process.
The study of transcription has far-reaching implications for fields such as genetics, medicine, and biotechnology. By exploring the mechanisms and regulation of transcription, we gain a deeper understanding of the molecular basis of life and the potential to develop novel therapeutic and biotechnological applications. As research continues to advance, the future holds exciting possibilities for uncovering new insights into transcription and its role in health and disease.
For further reading and resources on transcription and gene expression, consider exploring educational websites such as the National Center for Biotechnology Information (NCBI), which offers a wealth of information on molecular biology and genetics.
The Fascinating World Of The Trumpy Trout Commercial
Unlocking Managerial Success: Understanding The Three Essential Categories Of Skills
The Essential Guide To Understanding NBA Starting Lineups


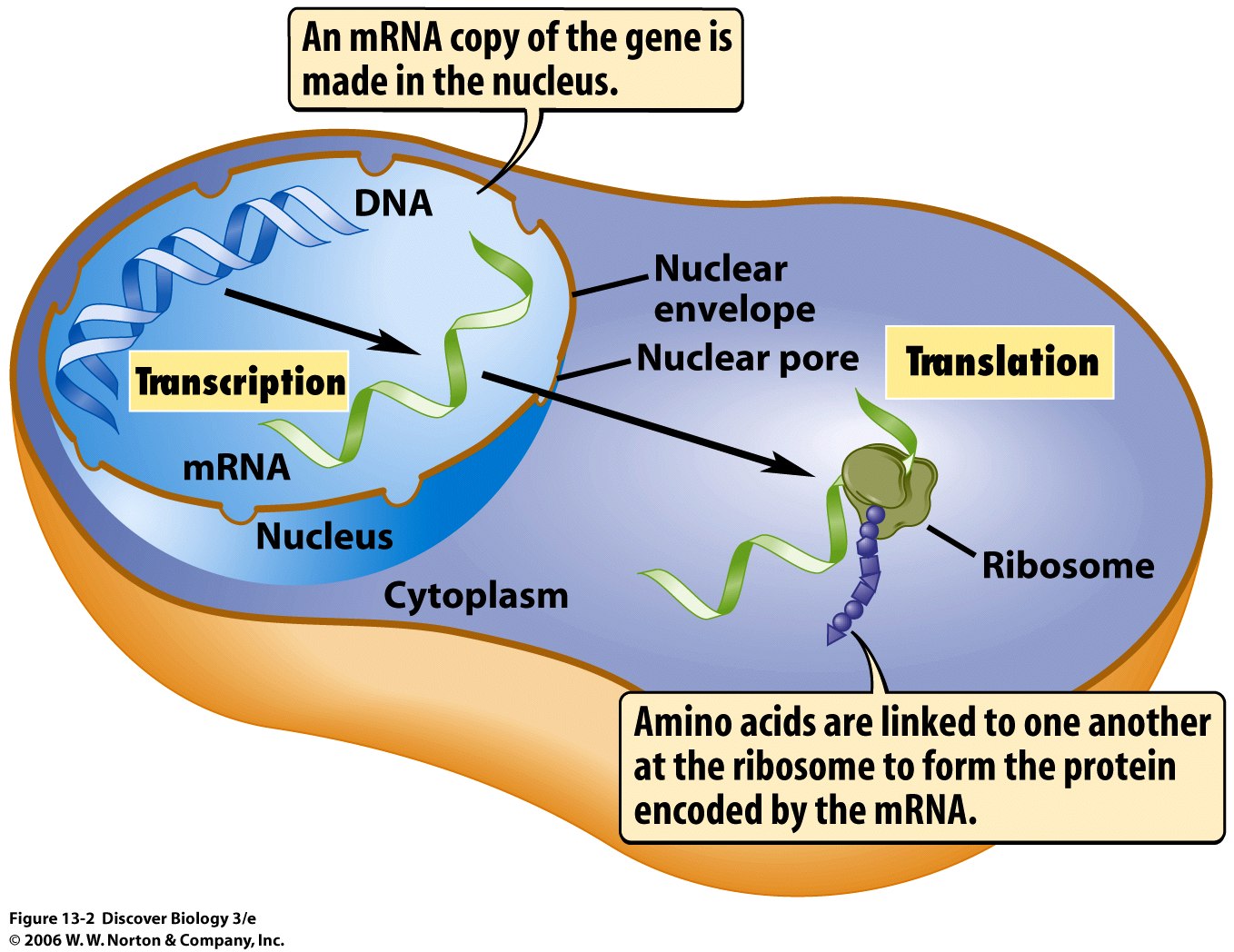