The universe has long been a subject of human curiosity and wonder, and at the heart of this fascination is the Big Bang theory. Originally proposed in the 20th century, the Big Bang theory posits that the universe began as an infinitely small, hot, and dense point approximately 13.8 billion years ago. From this singularity, the universe has been expanding, evolving, and cooling, giving rise to galaxies, stars, planets, and all forms of matter and energy. But as our understanding of the cosmos advances, questions arise: is the Big Bang still alive as the predominant explanation for our universe's origins? Or are there alternative theories that challenge its supremacy?
In recent years, new discoveries and technological advancements in astronomy and physics have provided fresh insights into the nature of the universe, prompting scientists to revisit and refine the Big Bang theory. Observations of cosmic microwave background radiation, the distribution of galaxies, and the behavior of dark energy and dark matter have all contributed to a deeper, more nuanced understanding of cosmic history. These developments raise intriguing questions about the Big Bang's validity, relevance, and adaptability in light of new evidence and theoretical advancements.
As we delve deeper into the complexities of the universe, it's essential to explore the various facets of the Big Bang theory, from its historical origins to its current standing in the scientific community. This article will examine the theory's strengths and weaknesses, consider the implications of recent discoveries, and discuss whether the Big Bang is still alive as a cornerstone of cosmology. Through a comprehensive analysis of scientific literature, expert opinions, and cutting-edge research, this article aims to provide a thorough and engaging overview of one of the most profound questions in science today.
Table of Contents
- Origin of the Big Bang Theory
- Evidence Supporting the Big Bang
- Cosmic Microwave Background Radiation
- Galaxy Distribution and Redshift
- Dark Matter and Dark Energy
- Alternative Theories
- Big Bang Theory Refinements
- The Role of Inflation
- Quantum Cosmology
- Multiverse Theory
- Current Standing in the Scientific Community
- Implications of Recent Discoveries
- Is the Big Bang Still Alive?
- Frequently Asked Questions
- Conclusion
Origin of the Big Bang Theory
The concept of the Big Bang can be traced back to the early 20th century when scientists began to grapple with the vastness of the universe and its origins. One of the earliest proponents of the idea was Georges Lemaître, a Belgian physicist and priest, who proposed the "hypothesis of the primeval atom" or the "cosmic egg" in 1927. Lemaître suggested that the universe was expanding, a revolutionary idea at the time, which laid the groundwork for the Big Bang theory.
In 1929, Edwin Hubble's observations provided the first empirical evidence for an expanding universe. By measuring the redshifts of distant galaxies, Hubble demonstrated that they were moving away from us, implying that the universe was indeed expanding. This observation supported Lemaître's hypothesis and challenged the then-dominant static universe model proposed by Albert Einstein, who later embraced the Big Bang theory.
The term "Big Bang" was coined by British astronomer Fred Hoyle during a 1949 radio broadcast, although he intended it as a pejorative term. Despite Hoyle's skepticism, the name stuck, and the theory gained traction as more evidence accumulated. Over the decades, the Big Bang theory has become the prevailing cosmological model, explaining the universe's origins and evolution with remarkable accuracy.
Evidence Supporting the Big Bang
The Big Bang theory is supported by a multitude of observational and theoretical evidence. One of the most compelling pieces of evidence is the cosmic microwave background (CMB) radiation, a faint glow left over from the early universe. Discovered in 1965 by Arno Penzias and Robert Wilson, the CMB provides a snapshot of the universe approximately 380,000 years after the Big Bang, when it had cooled enough for atoms to form and light to travel freely through space.
Another key piece of evidence is the abundance of light elements, such as hydrogen, helium, and lithium, observed throughout the universe. The Big Bang nucleosynthesis theory predicts the proportions of these elements based on the conditions present in the first few minutes after the Big Bang. Observations of these proportions align closely with theoretical predictions, bolstering the Big Bang theory's credibility.
The large-scale structure of the universe, including the distribution of galaxies and galaxy clusters, also supports the Big Bang model. Observations of galaxy redshifts, the stretching of light waves as galaxies move away from us, reveal an expanding universe consistent with Big Bang predictions. Furthermore, computer simulations of cosmic evolution based on the Big Bang theory have successfully replicated the observed large-scale structure of the universe.
Cosmic Microwave Background Radiation
The cosmic microwave background (CMB) radiation is one of the most significant discoveries supporting the Big Bang theory. It is the afterglow of the Big Bang, a relic radiation that fills the universe and provides critical insights into its early conditions. The CMB was first detected accidentally by Arno Penzias and Robert Wilson in 1965, leading to a Nobel Prize in Physics in 1978.
The CMB is a nearly uniform background of microwave radiation that permeates the cosmos at a temperature of approximately 2.7 Kelvin. Its uniformity and slight anisotropies, or variations, provide a wealth of information about the universe's initial conditions, its composition, and its subsequent evolution. Detailed measurements of the CMB by missions such as the Cosmic Background Explorer (COBE), the Wilkinson Microwave Anisotropy Probe (WMAP), and the Planck satellite have refined our understanding of the universe's age, geometry, and composition.
The CMB's temperature fluctuations, mapped with remarkable precision by these missions, reveal the seeds of cosmic structure. These anisotropies correspond to regions of slightly different densities in the early universe, which later grew into galaxies and galaxy clusters through gravitational collapse. The CMB thus serves as a powerful tool for testing cosmological models and constraining fundamental parameters of the universe.
Galaxy Distribution and Redshift
The distribution of galaxies and their redshifts provide crucial evidence for the Big Bang theory. Edwin Hubble's discovery of the redshift-distance relationship in 1929 demonstrated that galaxies are moving away from us, with more distant galaxies receding faster. This observation, known as Hubble's law, indicates that the universe is expanding, a key prediction of the Big Bang model.
Galaxy redshifts result from the expansion of space itself, causing the light waves emitted by galaxies to stretch and shift toward longer, redder wavelengths. This cosmological redshift is distinct from the Doppler effect, which arises from the motion of galaxies through space. The patterns of galaxy redshifts and their distribution across the sky reveal the large-scale structure of the universe, including the formation of galaxy clusters, superclusters, and cosmic voids.
Surveys of galaxy redshifts, such as the Sloan Digital Sky Survey (SDSS), have mapped the three-dimensional distribution of galaxies, providing a detailed view of the universe's structure. These observations align with predictions from the Big Bang model and support the theory's validity. The large-scale structure of the universe, characterized by filaments, walls, and voids, reflects the gravitational growth of initial density fluctuations imprinted in the cosmic microwave background.
Dark Matter and Dark Energy
Dark matter and dark energy are two enigmatic components of the universe that play crucial roles in the Big Bang theory and our understanding of cosmic evolution. Although they have not been directly observed, their effects are inferred through gravitational interactions and the universe's accelerated expansion.
Dark matter, which constitutes approximately 27% of the universe's mass-energy content, is believed to be composed of non-luminous, non-baryonic particles that do not interact with electromagnetic forces. Its presence is inferred from the gravitational effects on visible matter, such as the rotation curves of galaxies and the gravitational lensing of light from distant objects. Dark matter is essential for explaining the formation and evolution of galaxies and the large-scale structure of the universe.
Dark energy, accounting for about 68% of the universe's mass-energy content, is a mysterious force driving the accelerated expansion of the universe. Its existence was first inferred from observations of distant supernovae in the late 1990s, which revealed that the universe's expansion rate is increasing. Dark energy is often associated with the cosmological constant, a term introduced by Einstein, or with more exotic theories such as quintessence or modified gravity.
The inclusion of dark matter and dark energy in the Big Bang model has led to the development of the Lambda Cold Dark Matter (ΛCDM) cosmological model, which is the current standard model of cosmology. Although the nature of dark matter and dark energy remains unknown, their inclusion has led to a more comprehensive understanding of the universe's composition, evolution, and fate.
Alternative Theories
While the Big Bang theory is the dominant cosmological model, alternative theories have been proposed to explain the universe's origins and evolution. Some of these theories challenge the Big Bang's assumptions, while others attempt to address its limitations and unresolved questions.
One alternative is the steady-state theory, proposed by Fred Hoyle, Thomas Gold, and Hermann Bondi in 1948. This theory posits that the universe has no beginning or end and is in a constant state of expansion, with new matter continuously created to maintain a constant density. Although the steady-state theory was once a serious contender, it has been largely discredited due to the overwhelming evidence supporting the Big Bang, such as the cosmic microwave background radiation and the abundance of light elements.
The cyclic model, another alternative, suggests that the universe undergoes infinite cycles of expansion and contraction, with each cycle beginning with a Big Bang and ending in a Big Crunch. This model addresses some of the Big Bang's limitations, such as the initial singularity problem, but faces challenges in explaining observed phenomena like the CMB and the universe's accelerated expansion.
Other alternative theories include quantum cosmology, which attempts to describe the universe's origins using principles of quantum mechanics, and the multiverse hypothesis, which posits the existence of multiple, possibly infinite, universes with different physical laws. While these theories offer intriguing possibilities, they often lack empirical support and remain speculative.
Big Bang Theory Refinements
As our understanding of the universe has advanced, the Big Bang theory has undergone significant refinements to address its limitations and incorporate new discoveries. These refinements have strengthened the theory's explanatory power and aligned it with observed phenomena.
One major refinement is the incorporation of cosmic inflation, a rapid expansion of the universe that occurred within the first fraction of a second after the Big Bang. Proposed by Alan Guth in 1980, inflation addresses several problems with the original Big Bang model, such as the horizon and flatness problems. It also provides a mechanism for generating the initial density fluctuations that gave rise to the large-scale structure of the universe.
Another refinement is the incorporation of dark matter and dark energy, as discussed earlier. These components are essential for explaining the universe's observed structure and accelerated expansion. Their inclusion has led to the development of the ΛCDM model, which provides a comprehensive framework for understanding cosmic evolution.
Advances in observational technology, such as the detection of gravitational waves and the precise measurement of cosmic microwave background anisotropies, have further refined the Big Bang theory. These observations have provided new insights into the universe's early conditions, its geometry, and its fundamental parameters, leading to a more accurate and detailed picture of cosmic history.
The Role of Inflation
Cosmic inflation is a critical component of the refined Big Bang theory, addressing several key issues with the original model and providing a more complete understanding of the universe's early evolution. Inflation posits that the universe underwent a brief period of exponential expansion, growing by a factor of at least 1026 in less than 10-32 seconds.
One of the primary problems inflation addresses is the horizon problem, which arises from the observed uniformity of the cosmic microwave background. In the original Big Bang model, regions of the universe separated by vast distances should not have had time to come into thermal equilibrium, yet the CMB temperature is remarkably uniform across the sky. Inflation solves this problem by positing that the entire observable universe was once a small, causally connected region before undergoing rapid expansion.
Inflation also addresses the flatness problem, which concerns the universe's observed geometric flatness. In the absence of inflation, even slight deviations from flatness in the early universe would have grown over time, leading to a universe with a different geometry. Inflation's rapid expansion smooths out any initial curvature, resulting in the observed flatness.
Additionally, inflation provides a mechanism for generating the initial density fluctuations that seeded the formation of galaxies and large-scale structures. Quantum fluctuations during inflation are stretched to macroscopic scales, leaving imprints in the cosmic microwave background and the distribution of galaxies. These predictions have been confirmed by observations, lending strong support to the inflationary model.
Quantum Cosmology
Quantum cosmology is an area of research that seeks to understand the universe's origins and behavior using the principles of quantum mechanics. It aims to address the limitations of classical cosmology, particularly the initial singularity problem, where the laws of physics break down.
One approach within quantum cosmology is the concept of a quantum universe, where the universe's initial state is described by a wave function, akin to the wave functions of particles in quantum mechanics. This wave function provides a probabilistic description of the universe's possible configurations and histories, potentially eliminating the singularity problem.
The Hartle-Hawking no-boundary proposal is a prominent example of quantum cosmology. Proposed by James Hartle and Stephen Hawking, this model suggests that the universe has no initial boundary or singularity. Instead, it transitions smoothly from a quantum state to a classical state, similar to how a wave function collapses to a definite outcome in quantum mechanics.
Path integral quantum cosmology is another approach, where the universe's evolution is described by a sum over all possible histories, weighted by their probability amplitudes. This approach provides a framework for calculating the probability of different cosmological scenarios and exploring the implications of quantum mechanics on cosmic scales.
While quantum cosmology offers intriguing possibilities for understanding the universe's origins, it remains a speculative field with many open questions. The lack of empirical evidence and the challenges of reconciling quantum mechanics with general relativity are significant hurdles that researchers continue to address.
Multiverse Theory
The multiverse theory is a speculative concept that suggests the existence of multiple, possibly infinite, universes beyond our observable cosmos. These universes, collectively known as the multiverse, may have different physical laws, constants, and properties, leading to a vast array of possible realities.
Several hypotheses propose the existence of a multiverse, each arising from different theoretical frameworks. One such hypothesis is the inflationary multiverse, which suggests that different regions of space undergo different rates and durations of inflation, resulting in separate "bubble" universes with varying properties.
Another multiverse hypothesis arises from string theory, a theoretical framework that attempts to unify all fundamental forces and particles. In string theory, the landscape of possible solutions, known as the "string landscape," allows for a multitude of different vacuum states, each corresponding to a different universe with distinct physical characteristics.
The many-worlds interpretation of quantum mechanics offers yet another multiverse perspective. This interpretation posits that every quantum event results in a branching of the universe into multiple, non-communicating branches, each representing a different outcome of the event.
While the multiverse theory offers intriguing possibilities, it remains highly speculative and lacks empirical support. The challenge of testing and falsifying multiverse hypotheses is significant, as these universes are often beyond the reach of direct observation and experimentation. Nonetheless, the multiverse theory continues to inspire scientific inquiry and philosophical debate about the nature of reality.
Current Standing in the Scientific Community
The Big Bang theory remains the prevailing cosmological model within the scientific community, providing a comprehensive framework for understanding the universe's origins, evolution, and large-scale structure. Its success in explaining a wide range of observations, from the cosmic microwave background to the distribution of galaxies, has solidified its status as the cornerstone of modern cosmology.
Despite its prominence, the Big Bang theory is not without its challenges and open questions. The nature of dark matter and dark energy, the initial singularity problem, and the integration of quantum mechanics with general relativity are among the unresolved issues that continue to drive research and theoretical development.
Alternative theories and refinements, such as cosmic inflation and quantum cosmology, have been proposed to address these challenges and extend the Big Bang model's explanatory power. While some alternatives lack empirical support, they contribute to the ongoing dialogue and exploration of the universe's fundamental nature.
The scientific community remains committed to testing and refining cosmological models through observations and experiments. Advances in technology, such as next-generation telescopes and gravitational wave detectors, promise to provide new insights and data that will further shape our understanding of the universe's history and composition.
Implications of Recent Discoveries
Recent discoveries in astronomy and physics have significant implications for our understanding of the Big Bang theory and the universe's origins. These discoveries, driven by advances in observational technology and theoretical development, continue to refine and challenge our cosmological models.
One of the most significant discoveries is the detection of gravitational waves, ripples in spacetime caused by the acceleration of massive objects. The detection of these waves by the LIGO and Virgo collaborations has opened a new window for observing the universe and testing the predictions of general relativity. Gravitational wave astronomy offers the potential to probe the universe's earliest moments and explore phenomena such as black hole mergers and neutron star collisions.
Another important discovery is the detailed mapping of the cosmic microwave background anisotropies by the Planck satellite. These measurements have provided precise constraints on the universe's age, composition, and geometry, refining our understanding of cosmic inflation and the initial conditions of the universe.
Observations of the accelerated expansion of the universe have also led to new insights into dark energy and its role in cosmic evolution. These observations have prompted the development of new theoretical models and the exploration of modified gravity theories to explain the universe's behavior on large scales.
The search for dark matter particles in laboratory experiments and astrophysical observations continues to advance, with new detection methods and candidate particles being explored. The identification of dark matter's nature would have profound implications for our understanding of galaxy formation and cosmic structure.
Is the Big Bang Still Alive?
The question of whether the Big Bang is still alive as the predominant cosmological model is a subject of ongoing scientific exploration and debate. While the Big Bang theory has withstood numerous tests and challenges, its continued relevance depends on its ability to accommodate new discoveries and address unresolved questions.
The incorporation of cosmic inflation, dark matter, and dark energy has strengthened the Big Bang model, providing a more comprehensive framework for understanding cosmic evolution. The ΛCDM model, which includes these components, remains the standard model of cosmology, successfully explaining a wide range of observations.
However, unresolved issues, such as the nature of dark matter and dark energy, the initial singularity problem, and the integration of quantum mechanics with cosmology, highlight the need for continued research and theoretical development. Alternative theories, such as quantum cosmology and the multiverse hypothesis, offer intriguing possibilities but require further exploration and empirical support.
Ultimately, the Big Bang theory's continued vitality depends on its adaptability and ability to incorporate new evidence and insights. As observational technology advances and theoretical frameworks evolve, the scientific community remains committed to refining and testing our understanding of the universe's origins and evolution.
Frequently Asked Questions
1. What is the Big Bang theory?
The Big Bang theory is a cosmological model that describes the universe's origins and evolution. It posits that the universe began as an infinitely small, hot, and dense point approximately 13.8 billion years ago and has been expanding, evolving, and cooling ever since.
2. What evidence supports the Big Bang theory?
The Big Bang theory is supported by several key observations, including the cosmic microwave background radiation, the abundance of light elements, and the large-scale distribution of galaxies and their redshifts. These observations align with the predictions of the Big Bang model.
3. What is cosmic inflation?
Cosmic inflation is a theoretical refinement of the Big Bang model that proposes a rapid expansion of the universe within the first fraction of a second after the Big Bang. Inflation addresses several key problems with the original model and provides a mechanism for generating the initial density fluctuations that led to the formation of cosmic structures.
4. What are dark matter and dark energy?
Dark matter and dark energy are two mysterious components of the universe. Dark matter is a non-luminous form of matter that interacts gravitationally with visible matter, while dark energy is a force driving the accelerated expansion of the universe. Together, they account for approximately 95% of the universe's mass-energy content.
5. Are there alternative theories to the Big Bang?
Yes, several alternative theories have been proposed, including the steady-state theory, the cyclic model, quantum cosmology, and the multiverse hypothesis. While some of these theories offer intriguing possibilities, they often lack empirical support and remain speculative.
6. Is the Big Bang theory still the dominant cosmological model?
Yes, the Big Bang theory remains the prevailing cosmological model within the scientific community. Its success in explaining a wide range of observations and its adaptability to incorporate new discoveries have solidified its status as the cornerstone of modern cosmology.
Conclusion
The Big Bang theory has been a cornerstone of modern cosmology, providing a comprehensive framework for understanding the universe's origins, evolution, and large-scale structure. Its success in explaining a wide range of observations, from the cosmic microwave background to the distribution of galaxies, has solidified its status as the dominant cosmological model.
While the Big Bang theory is not without its challenges and open questions, its adaptability and ability to incorporate new discoveries and insights have ensured its continued relevance. The incorporation of cosmic inflation, dark matter, and dark energy has strengthened the model, providing a more comprehensive understanding of cosmic evolution.
As observational technology advances and theoretical frameworks evolve, the scientific community remains committed to refining and testing our understanding of the universe's origins and evolution. While alternative theories offer intriguing possibilities, the Big Bang theory's continued vitality depends on its ability to accommodate new evidence and address unresolved questions.
The exploration of the universe's mysteries is a testament to human curiosity and ingenuity. As we continue to push the boundaries of knowledge, the Big Bang theory remains a vital and dynamic area of scientific inquiry, guiding us in our quest to unravel the secrets of the cosmos.
Understanding The Oct 23 Zodiac: A Comprehensive Guide To Astrological Insights
The Ultimate Guide To Finding The Best Bra Without Underwire
Mark Herzlich: The Inspiring Journey Of A New York Giants Legend
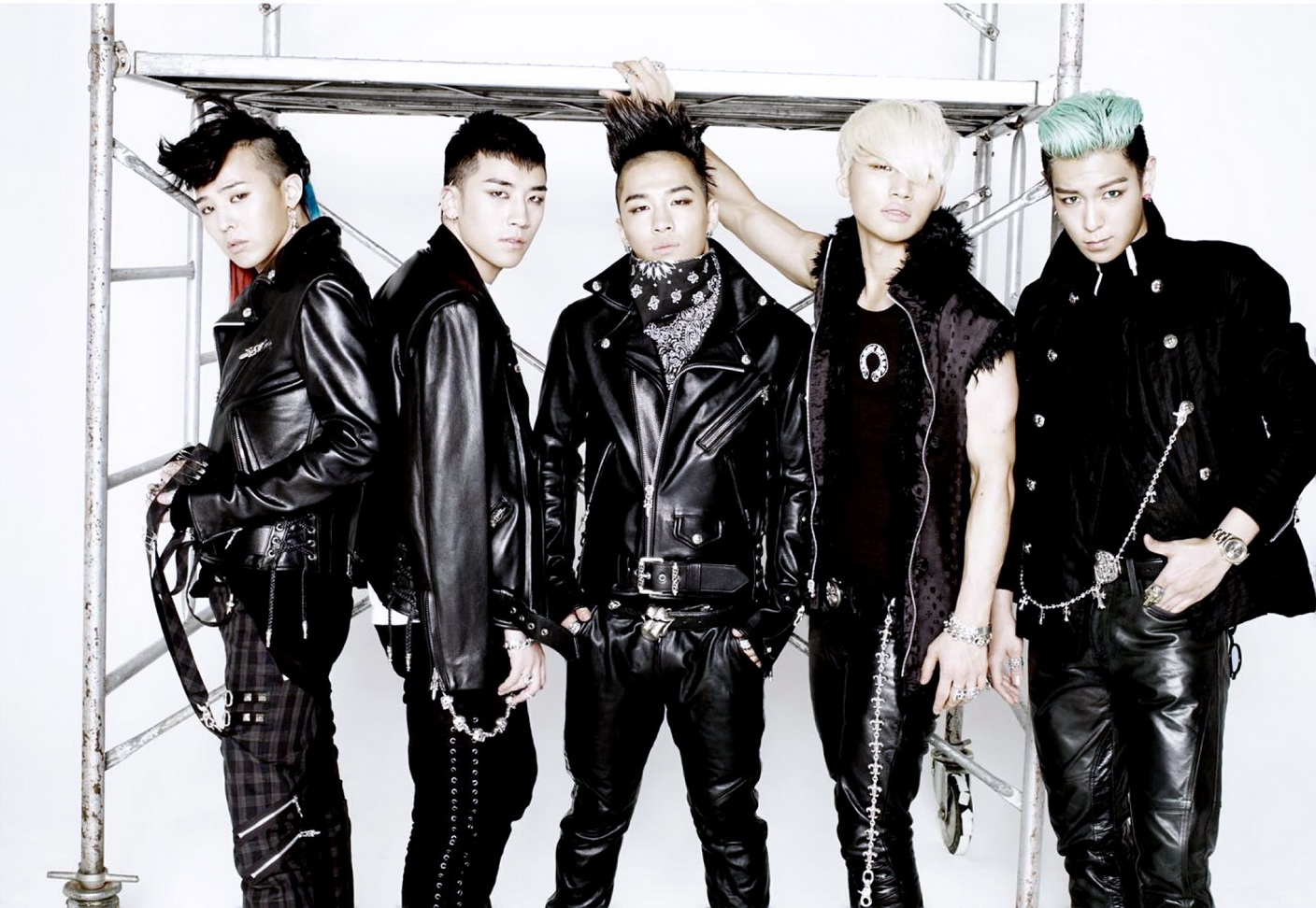
